Atmospheric chemistry modeling
-
As part of our efforts to investigate the effects of vegetation on photochemical air pollution, we develop updated chemical mechanisms to account for the interaction of the biogenic volatile organic compounds (BVOCs) from vegetation with anthropogenic air pollution. In parallel, we simulate the effect of the emitted BVOCs on photochemical air pollution, using mesoscale models such as WRF-Chem.

The figure presents the effect of anthropogenic emission on photochemical pollution based on a chemical mechanism that was developed in our group, using the CAABA/MECCA atmospheric chemistry model (Gabay and Tas, 2019). The results are presented for different fuels under photochemically VOC-limited conditions (VOC-limited) and photochemically NOx-limited conditions (NOx-limited) scenarios. Presented are, individually for each fuel, the difference (in percentage) between daytime mixing ratios, Leighton ratio (L), the ratio between NOx and total VOCs concentrations ([NOX]/[TVOC]) and [OH]/[HO ] vs. time, which were obtained for VOC-limited and NOX-limited and the corresponding background simulations (VOC-LIM-BG and NOX-LIM-BG, respectively in Gabay and Tas. (2019))
2

WRF-Chem simulations
Map of the three nested domain grids of WRF simulations in the Eastern Mediterranean (domain resolution: Domain 1 = 9 km, Domain 2 = 3 km , Domain 3 = 1 km). The WRF domain covers areas in southern Lebanon, Israel, West Syria, West Jordan, and South Egypt.
-
Our model simulations point out that reactive bromine and reactive iodine species can lead to ozone formation under high pollution of nitrogen oxides, such as nearby polluted coastal areas (Shechner and Tas, 2017)
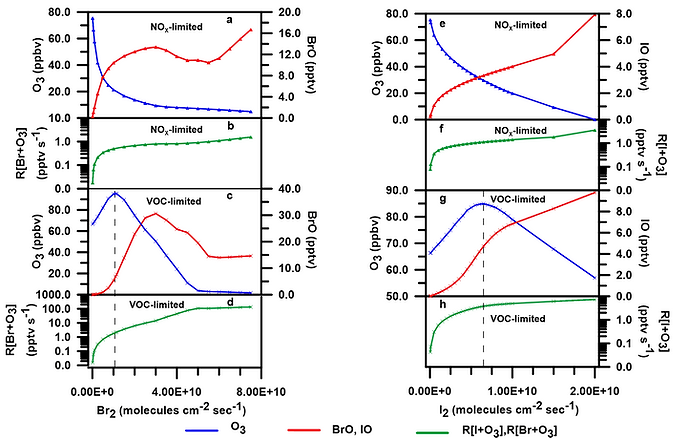
Response of ozone (O3) mixing ratios to increasing reactive halogen species activity. Each point on a curve represents the daily mean mixing ratios of a single simulation. The simulated daily average mixing ratios of O3 and XO (X = I or Br) are presented: (a and e) NOX-limited scenarios and (c and g) VOC-limited scenarios vs. the corresponding X2 (X = Br or I) emission fluxes. The daily average reaction rate (R) of O3 with the corresponding X is presented: (b and f) NOX-limited and (d and h) VOC-limited. Dashed lines indicate maximum Br2 or I2 flux for which an increase in daily average O3 mixing ratios occurred.
-
Literature review points to a dominant role of the involvement of photochemical pollution in atmospheric mercury oxidation. This was further supported using measurement-based box modeling simulations (Gabay and Tas, 2020)
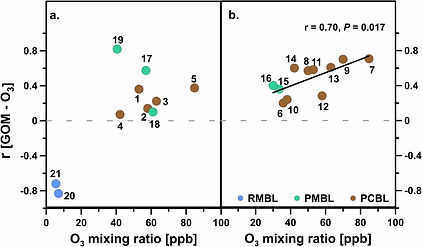
The Pearson correlation coefficient (r) of GOM-O3 as a function of the O3 mixing ratio at remote and polluted sites, taken from the literature (see detailed site information in Gabay and Tas, 2020). Blue, green and brown symbols represent remote marine boundary layer, polluted marine boundary layer and polluted continental boundary layer, respectively. Calculation of r used daily maximum (a) and hourly average (b) of GOM and O3, and is presented in both cases vs. daily maximum O3. The regression curve and parameters (r and P-value) of the correlation between the hourly average-based GOM–O3 correlation and daily maximum O3 mixing ratio are presented.